Laser Fusion Ignition: Putting Nuclear Fusion Breakthroughs into Perspective
This month, the media was abuzz with news that the US National Ignition Facility (NIF) had achieved a significant breakthrough in the quest for commercial nuclear fusion. Specifically, the announcement was that a net gain in fusion energy (Q) had been measured of approximately 1.5: for an input of 2.05 MJ, 3.15 MJ was produced.
>What was remarkable about this event compared to last year's 1.3 MJ production is that it demonstrates an optimized firing routine for NIF lasers, and that changes the way the Hohlraum - containing deuterium-tritium (D-T) fuel - is the targeted result in more efficient compression. In this Hohlraum, X-rays are produced which are used to compress the fuel. With sufficient pressure, the Coulomb barrier that usually prevents nuclei from approaching each other can be overcome, and this is fusion.
Based on preliminary results, it appears that a few percent of the D-T fuel has undergone meltdown. So the next question: does this really mean that we are closer to having commercial fusion reactors producing a lot of power?
Scientific communication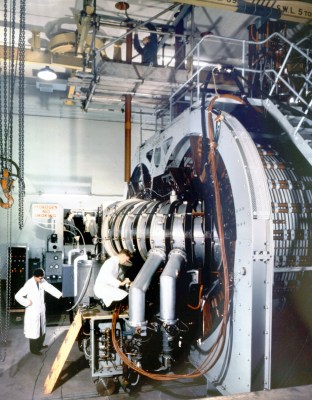
As the eternal boom continues, nuclear fusion is still a decade away, since its discovery a hundred years ago. What is unfortunately missing in much of the communication about research and development in fundamental physics is often a deeper understanding of what is going on and what the findings being reported mean. Since we are dealing with fundamental physics and moving boldly into new areas of plasma physics, high-temperature superconducting magnets, as well as exciting new areas in materials research, whatever we can do is provide a solid, informed estimate.
With the Z-pinch fusion reactors of the 1950s, it seemed like commercial fusion reactors were just a few years away. Simply pulse high currents through the plasma to induce fusion, harvest the energy and suddenly the much-vaunted nuclear fission reactors of the time already seemed like relics of yesteryear. With initially high Q figures reported for Z-pinch fusion reactors, headlines hit the headlines with absolute certainty that the UK would build the first fusion reactors, and the rest of the world would follow. p>
Later it was discovered that the measurements had been skewed, and neither the supposed fusion gain had been as staggering as reported, nor had anyone been aware of the seriousness of the plasma instabilities in this type of reactor which complicated their use. Only with the design of the Russian tokamak, which added an electromagnetic field around the plasma, did ...
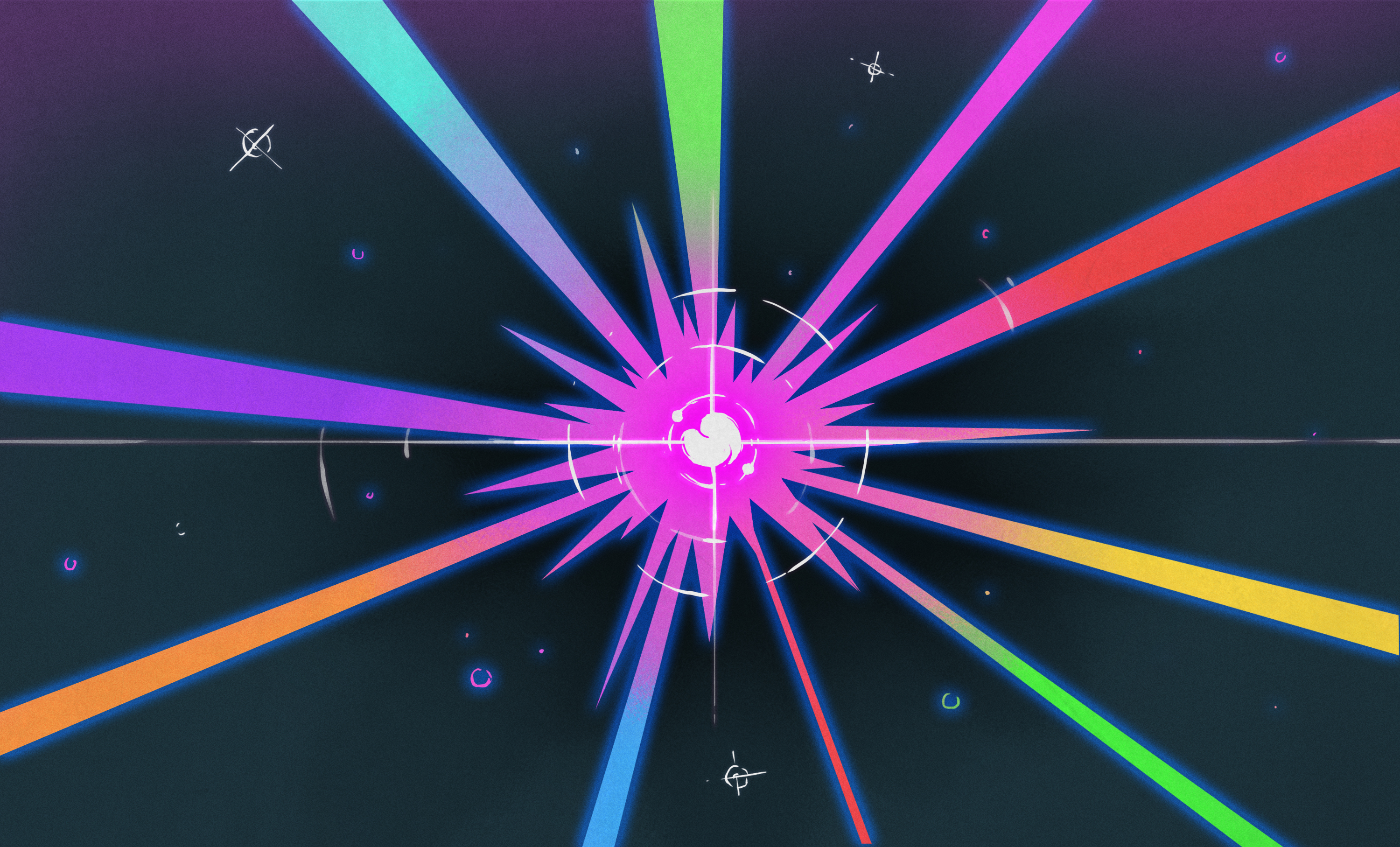
This month, the media was abuzz with news that the US National Ignition Facility (NIF) had achieved a significant breakthrough in the quest for commercial nuclear fusion. Specifically, the announcement was that a net gain in fusion energy (Q) had been measured of approximately 1.5: for an input of 2.05 MJ, 3.15 MJ was produced.
>What was remarkable about this event compared to last year's 1.3 MJ production is that it demonstrates an optimized firing routine for NIF lasers, and that changes the way the Hohlraum - containing deuterium-tritium (D-T) fuel - is the targeted result in more efficient compression. In this Hohlraum, X-rays are produced which are used to compress the fuel. With sufficient pressure, the Coulomb barrier that usually prevents nuclei from approaching each other can be overcome, and this is fusion.
Based on preliminary results, it appears that a few percent of the D-T fuel has undergone meltdown. So the next question: does this really mean that we are closer to having commercial fusion reactors producing a lot of power?
Scientific communication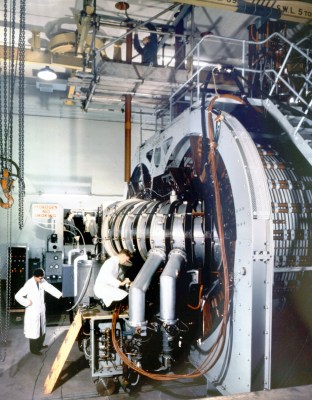
As the eternal boom continues, nuclear fusion is still a decade away, since its discovery a hundred years ago. What is unfortunately missing in much of the communication about research and development in fundamental physics is often a deeper understanding of what is going on and what the findings being reported mean. Since we are dealing with fundamental physics and moving boldly into new areas of plasma physics, high-temperature superconducting magnets, as well as exciting new areas in materials research, whatever we can do is provide a solid, informed estimate.
With the Z-pinch fusion reactors of the 1950s, it seemed like commercial fusion reactors were just a few years away. Simply pulse high currents through the plasma to induce fusion, harvest the energy and suddenly the much-vaunted nuclear fission reactors of the time already seemed like relics of yesteryear. With initially high Q figures reported for Z-pinch fusion reactors, headlines hit the headlines with absolute certainty that the UK would build the first fusion reactors, and the rest of the world would follow. p>
Later it was discovered that the measurements had been skewed, and neither the supposed fusion gain had been as staggering as reported, nor had anyone been aware of the seriousness of the plasma instabilities in this type of reactor which complicated their use. Only with the design of the Russian tokamak, which added an electromagnetic field around the plasma, did ...
What's Your Reaction?
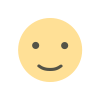
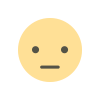
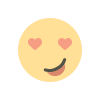
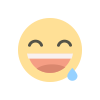
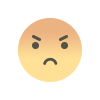
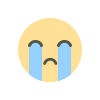
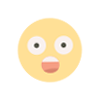